Aging is a multifactorial process – with “hallmarks” [1] including genomic instability and altered gene expression, epigenetic effects, telomere erosion, stem cell fatigue, proteostatic errors, senescence, compromised mitochondrial, lysosomal, and peroxisomal function, and disrupted communication networks, among others. Senescence, in particular, is critical as it is thought to constitute a major decision point for cells. With advancing age, cells accumulate sufficient damage and are stressed to the point that they must make a crucial decision – either transform and become cancerous, or pass into senescence.
The anti-tumor senescent state is one of continued metabolic activity, but no cell division. Unfortunately, accompanying the senescent phenotype is cellular release of assorted chemokines, growth factors, interleukins, and proteases. The ultimate effect of this collection of pro-inflammatory mediators is to corrupt the regional cell/tissue milieu. Ironically, this newly created environment is very conducive to cellular transformation and tumor development. Indeed, investigators in the field refer to the senescence-associated secretory phenotype as “the dark side of tumor suppression”[2].
What if the decision to pass into senescence could be maintained (and thus avoid cancerous transformation), but the senescent cell could somehow be destroyed? Would cancer be averted and cell/tissue integrity maintained? Could stem cell exhaustion be ameliorated and health span increased? Could aging, the ultimate risk factor for human disease, be brought under control – at least to some extent? While clear answers to these questions remain elusive, the idea of trying to destroy senescent cells – either through genetic means or specific drugs (called “senolytics”), is a major focus of researchers in the field of cellular aging.
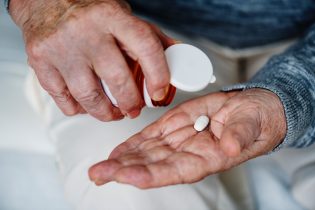
Dr. Van Deursen’s group at the Mayo Clinic College of Medicine developed a brilliant strategy of induced senescent cell suicide. In mouse models, they created a genetic background whereby cells would die the moment they expressed the senescence biomarker p16Ink4A. They showed both in progeroid[3] (i.e., prematurely aged) and wild-type[4] backgrounds, that targeted elimination of senescent cells improved the health of the animals by delaying, or preventing, impaired tissue function. Furthermore, in the wild-type background, the mice lived some 30% longer!
Instead of genetic approaches, investigators from Dr. Robbins’ group at the Scripps Research Institute screened for drugs that would selectively target senescent cells – while not affecting other cells. Interestingly, inhibitors of HSP90 were identified [5] as potent senolytics – with efficacy in both in vitro (i.e., cellular) and in vivo (i.e., whole animal) models. HSP90s are critical cellular chaperones, which facilitate protein folding and stabilize hundreds of molecules involved in a myriad of biochemical and metabolic pathways. The paper’s authors speculate that inhibiting HSP90’s anti-apoptotic (i.e., cell death)/pro-survival activities may be playing some role in selectively targeting senescent cells – a view consistent with the activity of other demonstrated senolytics[6] that are thought to reduce resistance to apoptosis.
How to partner senolytics with other drugs to maximize efficient elimination of senescent cells while limiting toxicity is an important focus going forward. Although the science is not quite there yet – the notion of clinical trials employing senoltyics or other modulators of cell senescence with the goal of thwarting aging’s effects and improving health span are not that far off.
SRT – September 2018
[1]C. Lopez-Otin et al., Cell. (2013) doi: 10.1016/j.cell.2013.05.039. (PMID: 23746838)
[2] J-P. Coppe et al., Ann Rev Pathol. (2010) doi: 10.1146/annurev-pathol-121808-102144 (PMID:20078217)
[3] D.J. Baker et al., Nature (2011) doi: 10.1038/nature10600. (PMID: 22048312)
[4] D.J. Baker et al., Nature (2016) doi: 10.1038/nature16932. (PMID: 26840489)
[5] H. Fuhrmann-Stroissnigg et al., Nat Commun. (2017) doi: 10.1038/s41467-017-00314-z. (PMID: 28871086)
[6]A. Hernandez-Segura et al., Trends in Cell Biology (2018) doi: 10.1016/j.tcb.2018.02.001 (PMID: 29477613)